APPLICATIONS
Energy materials: Perovskite solar cells
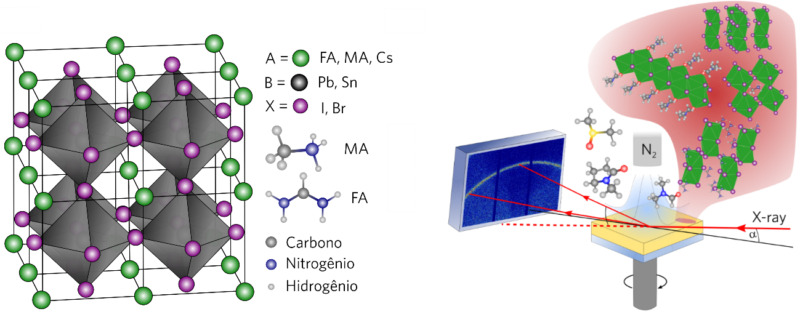
The dependence of humanity on energy for our current lifestyle is increasing year after year. Each time it is more critical the development of renewable sources like solar-based energy sources. Besides the extensive domain of silicon solar cells, the diversification of this sector is essential. The perovskite solar cells (PSCs) have gained attention between the emergent photovoltaic technologies due to fast growing efficiency, reaching conversions at the same level of silicon solar cells in just one decade. The perovskites used in PSCs are hybrid organic-inorganic perovskite (HOIP) with general formula ABX3, where A and B are cations and X an anion. The HOIP is composed of a mixture of organic cations such as methylammonium (MA+) and formamidinium (FA+) with small amounts of cesium (Cs+) on the A-site; Pb2+ on the B site; and iodide (I–) and bromide (Br–) anions on the X-site. Recently, we have investigated the first steps of perovskite crystallization during spin coating deposition and its stability at the UVX-LNLS source.1–4 The Carnaúba beamline at the Sirius source opens new possibilities to a deeper understanding of the perovskites at sub-micro and nanoscale by a diversity of techniques. These techniques will unveil the chemical composition, structure, morphology, and optical properties simultaneously. Moreover, in situ and operando approaches will allow the follow-up of materials changes close to the realistic solar cell application.
Szostak, R.; Marchezi, P. E.; Marques, A. dos S.; da Silva, J. C.; de Holanda, M. S.; Soares, M. M.; Tolentino, H. C. N.; Nogueira, A. F. Exploring the Formation of Formamidinium-Based Hybrid Perovskites by Antisolvent Methods: In Situ GIWAXS Measurements during Spin Coating. Sustain. Energy Fuels 2019, 3 (9). https://doi.org/10.1039/C9SE00306A.
Marchezi, P. E.; Therézio, E. M.; Szostak, R.; Loureiro, H. C.; Bruening, K.; Gold-Parker, A.; Melo, M. A.; Tassone, C. J.; Tolentino, H. C. N.; Toney, M. F.; Nogueira, A. F. Degradation Mechanisms in Mixed-Cation and Mixed-Halide CsxFA1−xPb(BryI1−y)3 Perovskite Films under Ambient Conditions. J. Mater. Chem. A 2020, 8 (18), 9302–9312. https://doi.org/10.1039/D0TA01201G.
Sutanto, A. A.; Szostak, R.; Drigo, N.; Queloz, V. I. E.; Marchezi, P. E.; Germino, J. C.; Tolentino, H. C. N.; Nazeeruddin, M. K.; Nogueira, A. F.; Grancini, G. In Situ Analysis Reveals the Role of 2D Perovskite in Preventing Thermal-Induced Degradation in 2D/3D Perovskite Interfaces. Nano Lett. 2020, 20 (5), 3992–3998. https://doi.org/10.1021/acs.nanolett.0c01271.
Szostak, R.; Sanchez, S.; Marchezi, P. E.; Marques, A. S.; Silva, J. C.; Holanda, M. S.; Hagfeldt, A.; Tolentino, H. C. N.; Nogueira, A. F. Revealing the Perovskite Film Formation Using the Gas Quenching Method by In Situ GIWAXS: Morphology, Properties, and Device Performance. Adv. Funct. Mater. 2021, 31 (4), 2007473. https://doi.org/10.1002/adfm.202007473.
Electrochemistry
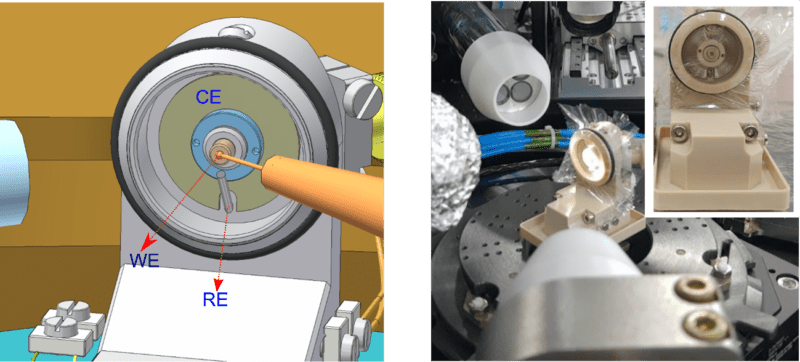
Electrochemistry is undeniably important in modern-day society as it impacts directly major technological, and economic sectors such as the environment, energy storage and conversion, biology, industry segments, and many others. In this context, a miniaturized electrochemical cell (Fig.1) was designed to attend in situ measurements of electrochemical systems using advanced synchrotron techniques in reflection mode. To guarantee versatility to the experiments, the cell was designed to be compatible with dozens of working electrodes (WE) and counter electrode (CE) types, and it is also equipped with a standard Ag/AgCl reference electrode (RE). In terms of applications, for example, it will be possible to perform BCDI (Bragg coherent diffraction imaging) experiments to track changes in the morphology and map the strain, a parameter that is well-known to influence the electrocatalytic behavior of materials[1]. In addition, elemental composition and the oxidation states of operando electrochemical processes may be determined by 2D nanofluorescence maps (nano-XRF), and spectromicroscopy experiments of X-ray absorption (nano-XAS).
Bragg Coherent Diffraction Imaging for In Situ Studies in Electrocatalysis. Rafael A. Vicente, Itamar T. Neckel, Subramanian K. R. S. Sankaranarayanan, José Solla-Gullon, and Pablo S. Fernández ACS Nano 2021 15 (4), 6129-6146, DOI: 10.1021/acsnano.1c01080.
Systemic effect of manganese in-vivo and in-vitro on tumor progression
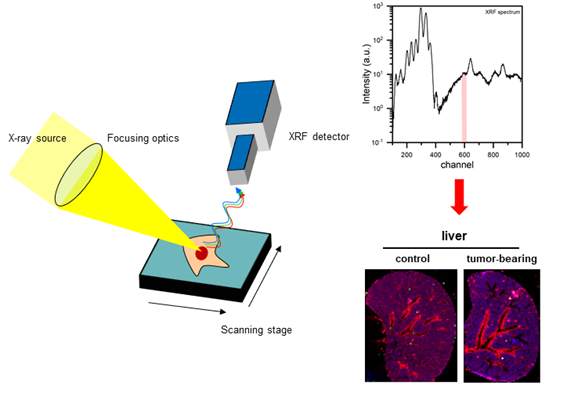
Cell migration is a relevant aspect of cancer, it participates in tumor progression from the very early steps of tumor microenvironment formation with the recruitment of local cells and the arrival of distant cells. Integrins are central molecules in migration connecting the extracellular matrix with the cytoskeleton. They mediate tumor microenvironment formation and arrival of inflammatory and metastatic cells into the healthy microenvironment. Therefore, understanding the mechanisms of integrin activation is essential for the study of tumor progression. Integrins are modulated by divalent cations that bind to distinct sites and regulate its function. Divalent cations are important for stabilizing integrin structure and modulating integrin-binding to its ligand, either enhancing or suppressing said binding. Specific concentrations of Ca2+ usually present an inhibitory effect, while Mn2+ enhances integrin-ligand binding by shifting integrins into high-affinity conformation. The role of metals in cancer progression remains to be further investigated. We recently investigate tumor progression from an underexplored point of view: metals modulation by the primary tumor and its systemic influence. Among several methods needed for this study, we used Synchrotron Radiation X-ray Fluorescence Microscopy (XRFM) to investigate the manganese distribution in control and tumor-bearing of different mice tissues in-vitro conditions. Our findings highlight manganese as a relevant character in tumor progression, participating in enhanced tumor cell migration and forming manganese-rich niches in primary tumors and distant organs. The CARNAÚBA beamline at the LNLS-Sirius source opens the possibility of investigating the accumulation of manganese in these niches with even much more spatial resolution (sub-micron and nanometric range) shedding more light on the role of this metal in tumor progression.
Stelling MP, Soares MA, Cardoso SC, Motta JM, Abreu J C, Antunes M J M, Freitas VG, Moraes J A, Castelo-Branco, MTL, Pérez CA, Pavão MSG. Manganese systemic distribution is modulated in vivo during tumor progression and affects tumor cell migration and invasion in vitro. Sci Rep 11, 15833, 2021. https://doi.org/10.1038/s41598-021-95190-5.
Photonics: Luminescent materials
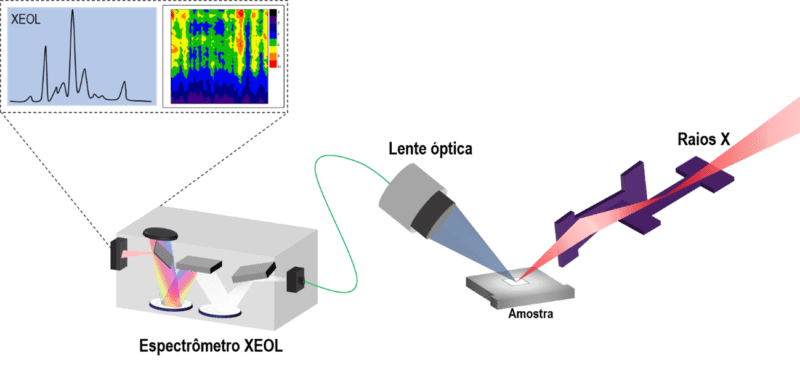
The development of photonic sources has boosted several technological sectors, ranging from medicine, with advanced imaging and therapy procedures such as the theranostic technique; to agriculture with the development of optical biomarkers to understand the nutrition uptake in plants. Thus, luminescent area significant for the development of optoelectronic devices, data transmission and storage, lighting sources, radiation detectors, imaging systems, light-emitting nanoparticles that can be functionalized and directed to a particular application, etc.
To understand the electronic structure and mechanisms associated with optical emission in this type of material, it is essential the use of techniques such as X-ray excited optical luminescence (XEOL), X-ray absorption, fluorescence, and diffraction, as well as imaging by several contrasts arising from the interaction of X-rays. In the Carnaúba beamline, besides having a multi-technical approach, the optical processes can be investigated through XEOL technique and with an X-ray nanoprobe (up to 30 nm), which will allow exploring several aspects such as chemical speciation, radiation hardness, distribution of emitting centers, etc., with high spectral and spatial resolution, opening new opportunities in the development and study of advanced photonic sources.
MINO, Lorenzo et al. Materials characterization by synchrotron x-ray microprobes and nanoprobes. Reviews of Modern Physics, v. 90, n. 2, p. 025007, 2018.
TEIXEIRA, V. C. et al. Luminescent properties of Li (Ga1-xCrx) 5O8 (LGCO) phosphors. Ceramics International, v. 46, n. 10, p. 15779-15785, 2020.
SEGURA-RUIZ, Jaime et al. Spatially and Time-Resolved Carrier Dynamics in Core–Shell InGaN/GaN Multiple-Quantum Wells on GaN Wire. Nano letters, v. 21, n. 22, p. 9494-9501, 2021.
WU, Y.-H. et al. Visualizing the valence states of europium ions in Eu-doped BaAl2O4 using X-ray nanoprobe mapping. Journal of Synchrotron Radiation, v. 29, n. 2, 2022.
Microfluidics
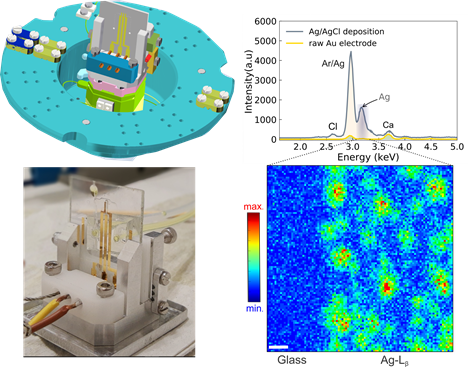
It is well known that microfluidics is a rapidly growing field attracting billions of dollars in investment every year in several strategical areas like medicine, materials science, biology, and chemistry, in which the understanding and development of new materials and/or complex systems is fundamental. Following the constant evolution of the microscopy techniques, which includes nowadays synchrotron-based techniques, that also might explore the highly coherent beams in fourth generation synchrotrons, we developed a microfluidic device to investigate structural, eletronic and optical properties of materials at multiple scales [1] in situ/operando. It is a polyester/glass sealed microfluidic device well-suited to combine with analytical X-ray techniques. Standard redox reactions probed the device’s throughput under electrochemical measurements, specifically cyclic voltammetry. Lastly, the 2D nanofluorescence experiments conducted at the Carnaúba beamline on pristine and silver modified gold electrodes revealed a device highly transparent to the X-rays as designed, and ready to be employed in experiments involving liquid sample environment. Overall, the device exhibits an exquisite chemical resistance to organic solvents, and its efficiency in the presence of biological samples (proteins) is remarkable.
Neckel, I.T., de Castro, L.F., Callefo, F. et al. Development of a sticker sealed microfluidic device for in situ analytical measurements using synchrotron radiation. Sci Rep 11, 23671 (2021). https://doi.org/10.1038/s41598-021-02928-2.
Nanopaleontology
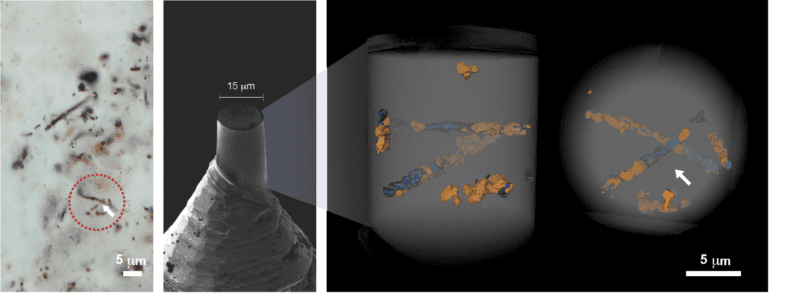
The study of the record of life on Earth, from its beginnings to the evolution to more complex forms that we know today, can be extensively investigated at its ultrastructural levels. With the application of the available techniques in the Carnauba beamline, by using the nanometric focus, structures previously not reached by the resolution limit (such as microfossils, biominerals and other potentially preserved biotic characters) can now be explored with a high level of compositional, structural and morphological details. Paleontological materials (or materials whose biogenicity is to be investigated) can be explored with a multi-technical and multi-scale approach combining the techniques available on the beamline. Examples are the application of XRD for mineralogy determination; XANES for evaluating the oxidation states of the elements; compositional analysis by XRF (punctual spectra or mapping); XEOL to obtain luminescence spectra and pticography for 2D and 3D imaging. Paleoenvironmental details about the fossils’ context, preservation mode (taphonomy), diagenetic details (changes that occurred during the fossil’s history) as well as information regarding the biogenicity of the material can be revealed. With this, Paleontology is elevated to a science to be explored at the nanoscale.
Maldanis, L., Hickman-Lewis, K., Verezhak, M., Gueriau, P., Guizar Sicairos, M., Jaqueto, P., Trindade, R.I.F., Rossi, A.L., Berenguer, F., Westall, F., Bertrand, L., Galante, D. 2020. Nanoscale 3D quantitative imaging of 1.88 Ga Gunflint microfossils reveals novel insights into taphonomic and biogenic characters. Scientific Reports, v. 10, p. 8163. Doi: https://doi.org/10.1038/s41598-020-65176-w
Callefo, F., Ricardi-Branco, F., Hartmann, G.A., Galante, D., Rodrigues, F., Maldanis, L., Yokoyama, E., Teixeira, V.C., Noffke, N., Bower, D.M., Bullock, E.S., Braga, A.H., Coaquira, J.A.H., Fernandes, M.A. 2019. Evaluating iron as a biomarker of rhythmites – An example from the last Paleozoic ice age of Gondwana. Sedimentary Geology, v. 383: 1-15. Doi: 10.1016/j.sedgeo.2019.02.002.